Gravitational Waves
Gravitational waves are disturbances in the curvature of spacetime, generated by accelerated masses, that propagate as waves outward from their source at the speed of light. They were proposed by Henri Poincaré in 1905 and subsequently predicted in 1916 by Albert Einstein on the basis of his general theory of relativity. Gravitational waves transport energy as gravitational radiation, a form of radiant energy similar to electromagnetic radiation. Newton's law of universal gravitation, part of classical mechanics, does not provide for their existence, since that law is predicated on the assumption that physical interactions propagate instantaneously (at infinite speed) – showing one of the ways the methods of classical physics are unable to explain phenomena associated with relativity.
Gravitational-wave astronomy is a branch of observational astronomy that uses gravitational waves to collect observational data about sources of detectable gravitational waves such as binary star systems composed of white dwarfs, neutron stars, and black holes; and events such as supernovae, and the formation of the early universe shortly after the Big Bang.
In 1993, Russell A. Hulse and Joseph H. Taylor, Jr. received the Nobel Prize in Physics for the discovery and observation of the Hulse-Taylor binary pulsar, which offered the first indirect evidence of the existence of gravitational waves.
On 11 February 2016, the LIGO and Virgo Scientific Collaboration announced they had made the first direct observation of gravitational waves. The observation was made five months earlier, on 14 September 2015, using the Advanced LIGO detectors. The gravitational waves originated from the merging of a binary black hole system. After the initial announcement the LIGO instruments detected two more confirmed, and one potential, gravitational wave events. In August 2017, the two LIGO instruments and the Virgo instrument observed a fourth gravitational wave from merging black holes, and a fifth gravitational wave from a binary neutron star merger. Several other gravitational wave detectors are planned or under construction.
In 2017, the Nobel Prize in Physics was awarded to Rainer Weiss, Kip Thorne and Barry Barish for their role in the direct detection of gravitational waves.
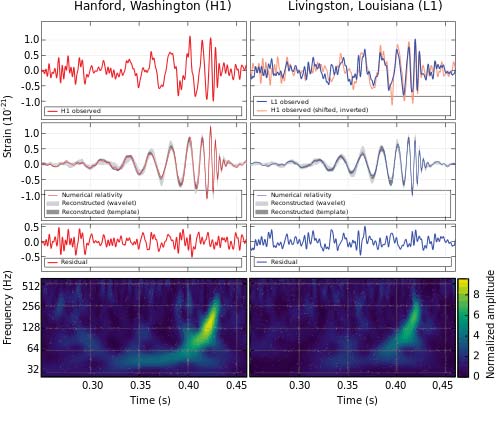
(B. P. Abbott et al. (LIGO Scientific Collaboration and Virgo Collaboration)
Gravitational Wave Observatories
Laser Interferometer Gravitational-Wave Observatory (LIGO)
The Laser Interferometer Gravitational-Wave Observatory (LIGO) is a large-scale physics experiment and observatory to detect cosmic gravitational waves and to develop gravitational-wave observations as an astronomical tool. Two large observatories were built in the United States with the aim of detecting gravitational waves by laser interferometry. These can detect a change in the 4 km mirror spacing of less than a ten-thousandth the charge diameter of a proton.
The initial LIGO observatories were funded by the National Science Foundation (NSF) and were conceived, built and are operated by Caltech and MIT. They collected data from 2002 to 2010 but no gravitational waves were detected.
The Advanced LIGO Project to enhance the original LIGO detectors began in 2008 and continues to be supported by the NSF, with important contributions from the United Kingdom's Science and Technology Facilities Council, the Max Planck Society of Germany, and the Australian Research Council. The improved detectors began operation in 2015. The detection of gravitational waves was reported in 2016 by the LIGO Scientific Collaboration (LSC) and the Virgo Collaboration with the international participation of scientists from several universities and research institutions. Scientists involved in the project and the analysis of the data for gravitational-wave astronomy are organized by the LSC, which includes more than 1000 scientists worldwide, as well as 440,000 active Einstein@Home users as of December 2016.
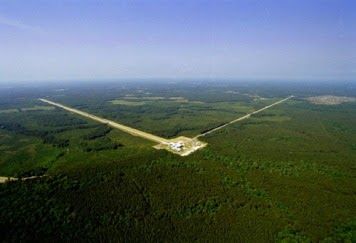
Observations are made in "runs". As of December 2019, LIGO has made 3 runs, and made 50 detections of gravitational waves. Maintenance and upgrades of the detectors are made between runs. The first run, O1, which ran from 12 September 2015 to 19 January 2016, made the first 3 detections, all were black hole mergers. The second run, O2, which ran from 30 November 2016 to 25 August 2017, made 8 detections, 7 black hole mergers, and the first neutron star merger. The third run, O3 began on 1 April 2019; it is divided (so far) into O3a, from 1 April to 30 September 2019, and O3b, from 1 November 2019 to December 2019. It has made 39 detections so far.
Advanced Virgo is an interferometric detector of gravitational waves hosted by the European Gravitational Observatory (EGO) near Pisa, in Italy. It can measure gravitational waves in a wide frequency range, from 10 Hz to 10000 Hz. Advanced Virgo is a laser interferometer with perpendicular, 3 km long arms and suspended mirrors. This is the configuration presently adopted in Advanced Virgo, during the third observation run (named O3, lasting 1 year from April 2019 to April 2020) performed jointly with the two Advanced LIGO detectors in the US and KAGRA in Japan.
Global Rapid Advanced Network Devoted to the Multi-messenger Addicts (GRANDMA)
An international scientific collaboration of astrophysicists that work on gravitational wave (GW) astronomy, utilizing crowdsourcing and volunteer astronomers to assist in research. GRANDMA utilizes a network of robotically controlled telescopes with both photometry and spectrometry capabilities for time-domain astronomy.
Kilonova Catcher is focused on the optical followup of any gravitational wave event that is detected by the currently operating gravitational wave observatories, specifically to catch the kilonova emissions emerging from the coalescence of two neutron stars bound in a compact binary system.
Multi-messenger astronomy is astronomy based on the coordinated observation and interpretation of disparate "messenger" signals. Interplanetary probes can visit objects within the Solar System, but beyond that, information must rely on "extrasolar messengers". The four extrasolar messengers are electromagnetic radiation, gravitational waves, neutrinos, and cosmic rays. They are created by different astrophysical processes, and thus reveal different information about their sources.
The main multi-messenger sources outside the heliosphere are expected to be compact binary pairs (black holes and neutron stars), supernovae, irregular neutron stars, gamma-ray bursts, active galactic nuclei, and relativistic jets. Detection from one messenger and non-detection from a different messenger can also be informative.
Electromagnetic observations of the sky have been the basis for study of the universe for millennia. Cosmic ray studies are now entering their second century, the first neutrinos from astrophysical sources were identified three decades ago, and gravitational waves were detected five years ago. Detection of these messengers are now common. In the near future, multimessenger detections will become routine. Success in the multimessenger era will require coordinated multimessenger and multiwavelength follow-ups. In particular, this will require 1) sensitive coverage of the non-electromagnetic messengers, 2) full coverage of the electromagnetic spectrum with either quick-response observations or broad and deep high-cadence surveys, and 3) improved collaboration, communications, and notification of multimessenger detection activities. This may represent a unique opportunity for the AAVSO to contribute to this rapidly growing field.
Astrophysical observations now include several messengers. Photons are the carriers of electromagnetic (EM) radiation. These are observed from radio wavelengths up to very high energy gamma rays. The EM spectrum covers more than twenty decades in energy. Gravitational waves (GW) are spacetime ripples emitted from systems with an accelerating quadrupole moment. Detectable GWs are expected to be emitted from binary systems of white dwarfs (WD), neutron stars (NS), and black holes (BH), and core-collapse supernovae (CCSN). Neutrinos are the lightest particles and are produced in weak nuclear interactions. In astrophysical processes MeV (million electron volt) neutrinos are produced in core-collapse events while high energy (TeV-PeV) neutrinos originate from supernova remnants or relativistic jets from active galactic nuclei. Cosmic rays are high energy charged particles such as protons or atomic nuclei. Heavy nuclei are formed and released in explosive nucleosynthesis and achieve high kinetic energies from natural particle accelerators (synchrotron radiation).
One very important electromagnetic messenger is radio frequency information detected by radio telescopes. There are several radio telescope observatories around the world that are doing research as part of multimessenger astrophysics, These include: